Section 41.1 Faraday's Experiments
In 1820 Hans Christian Øersted of Denmark published his finding that electric currents have magnetic effects. Here is a web link to a demo of that effect, Demo-site, where you will see a magnetic needle deflect when current passes through a metallic rod.
The discovery by Øersted inspired many other scientists, including French physicists, Jean-Baptiste Biot, Félix Savart, and André-Marie Ampère who very quickly found the basic laws governing magnetism associated with electric currents.
Ampere also demonstrated that wires carrying currents exerted force on each other. These findings brought great excitement to the scientific community in 1820s since they provided definitive experimental evidence of the close connections between electricity and magnetism - the two fields that had been hitherto separate and distinct from each other.
Soon people began to wonder if a magnetic field would produce an electric current as well and many ideas were tested. Most of these experiments didn't go anywhere. For instance, strong magnets next to a wire failed to produce any detectable current in the wire. Even the magnetic field produced by large steady currents in a wire failed to cause any current in a loop of wire next to the first wire. We call these experiments null experiments since the result is zero. They are illustrated in Figure 41.1.1.
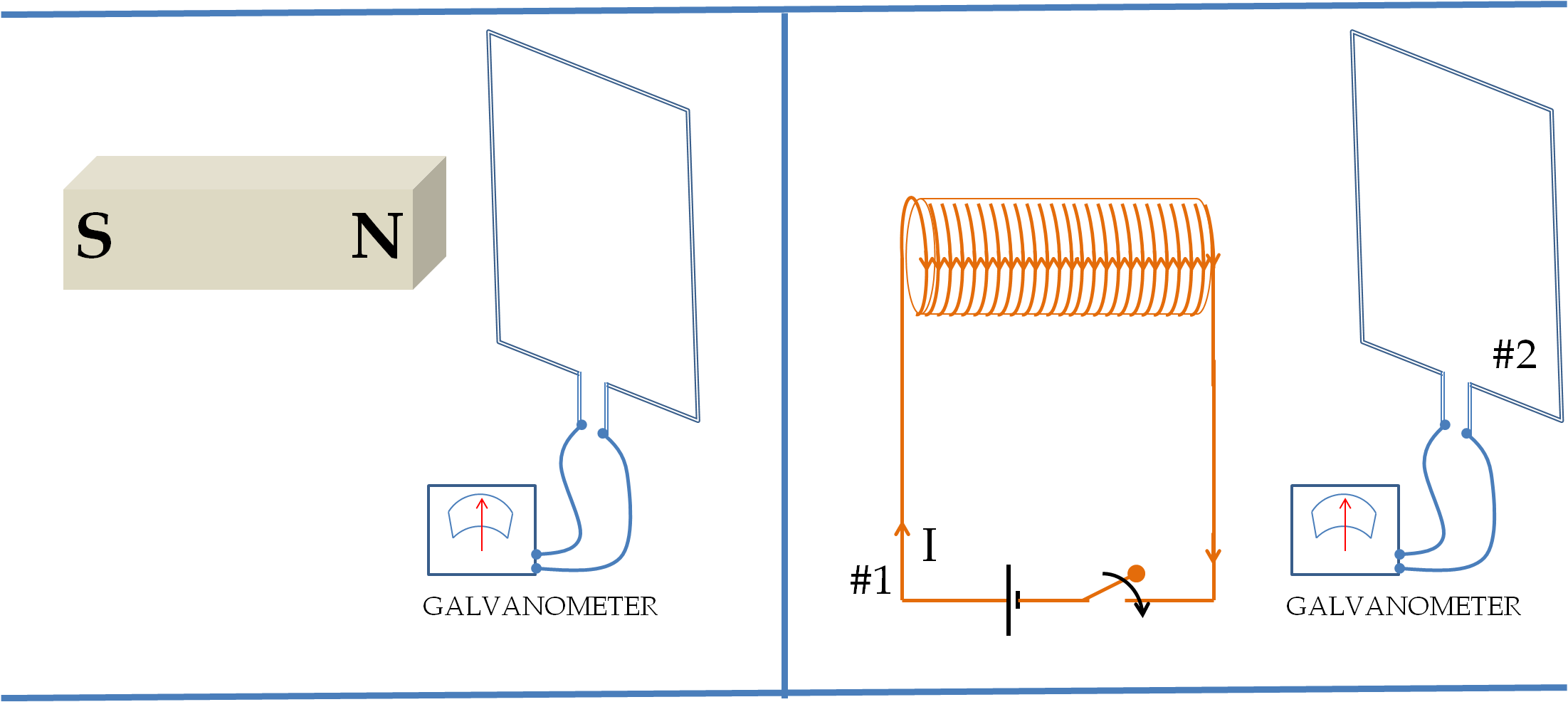
Moving Magnet Near a Metallic Loop
Around 1831 Michael Faraday found the missing link in these studies. He discovered that the electric effect of a magnetic field required that something must be changing with time. He observed that when a magnet is moving near a loop of wire a current is detected in the wire, but when the magnet stopped moving, there was no current in the wire as illustrated in Figure 41.1.2.
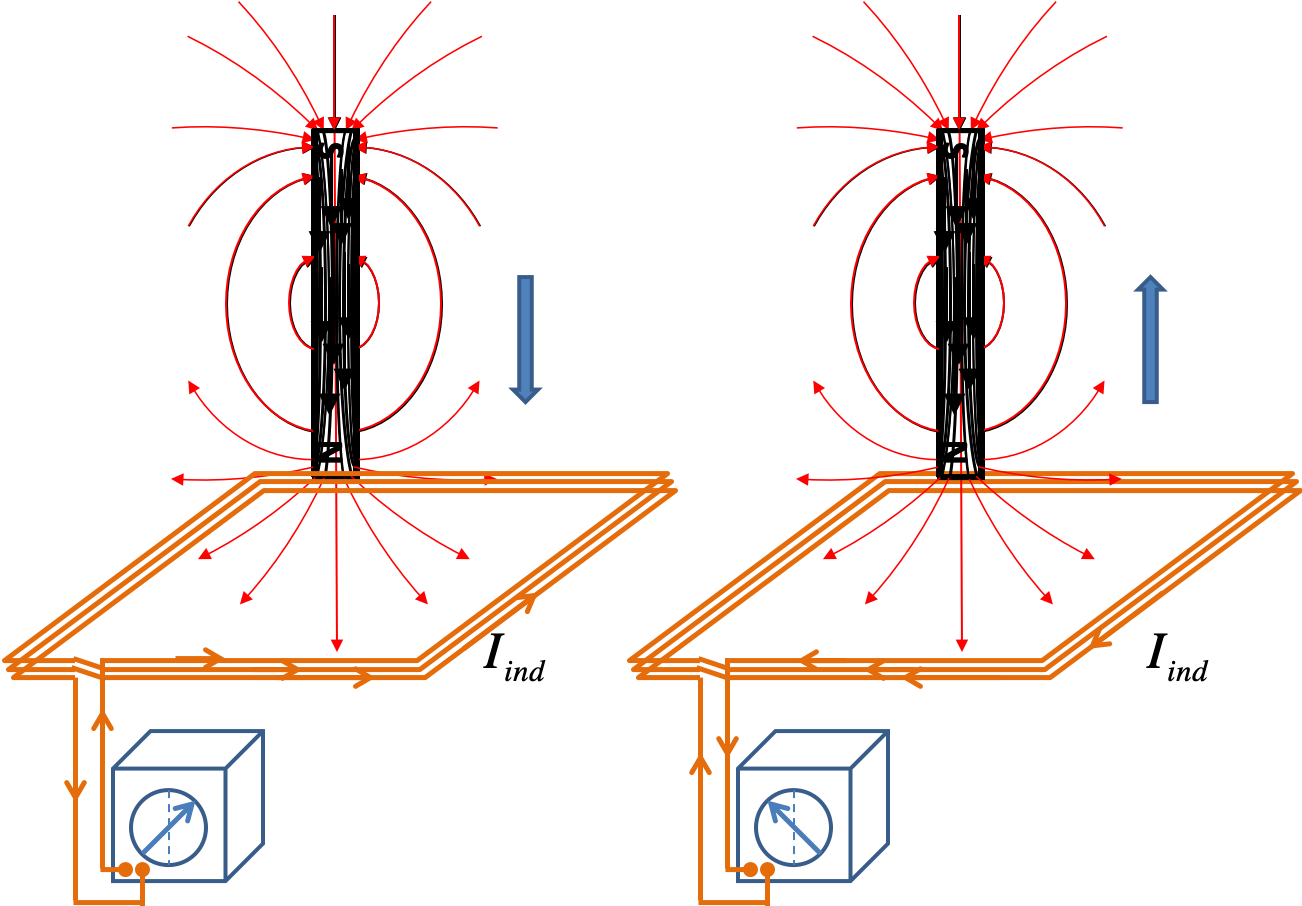
The direction of induced current is more easily seen by an application of Lenz's law. Lenz's law says induced current is in such as direction that its magnetic field opposes change in magnetic field through the loop occuring by whatever is changing in that loop's environment. We will practice Lenz's law later in the chapter. Here, you should see able to verify the induced current directions in each picture.
Moving a Metallic Loop Near a Magnet
Similarly, as illustrated in Figure 41.1.3, if a loop of wire is moving, e.g., if you are shaking around a loop of wire, in a space where there is some nonzero magnetic field, then a current will be detected in the wire during the time when the wire is moving but no there would be no current when you stop the motion of the wire.
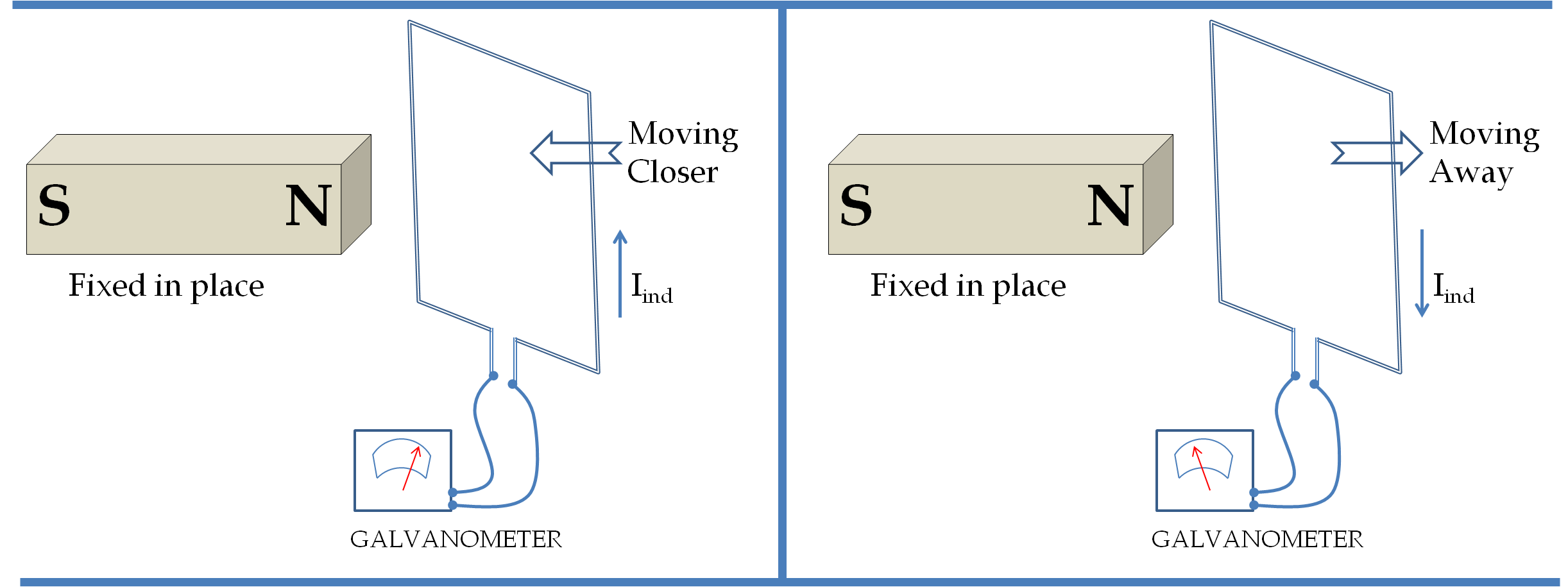
Changing Current in a Circuit near a Metallic Loop
Since magnetic field is also present near a current carrying wire, you can replace magnet in the above experiments and work with two wires, through one of which you pass some current by connecting it to a power source. When current in the wire with the power source is changing, then, simultaneously a current is detected in the other wire even though there is no apparent current source in the second wire. But when current in the first wire is not changing, then there is no current in the second wire as illustrated in Figure 41.1.4. In all these situations, we say that currents are induced whenever magnetic field through the loop, i.e., magnetic flux, is changing.
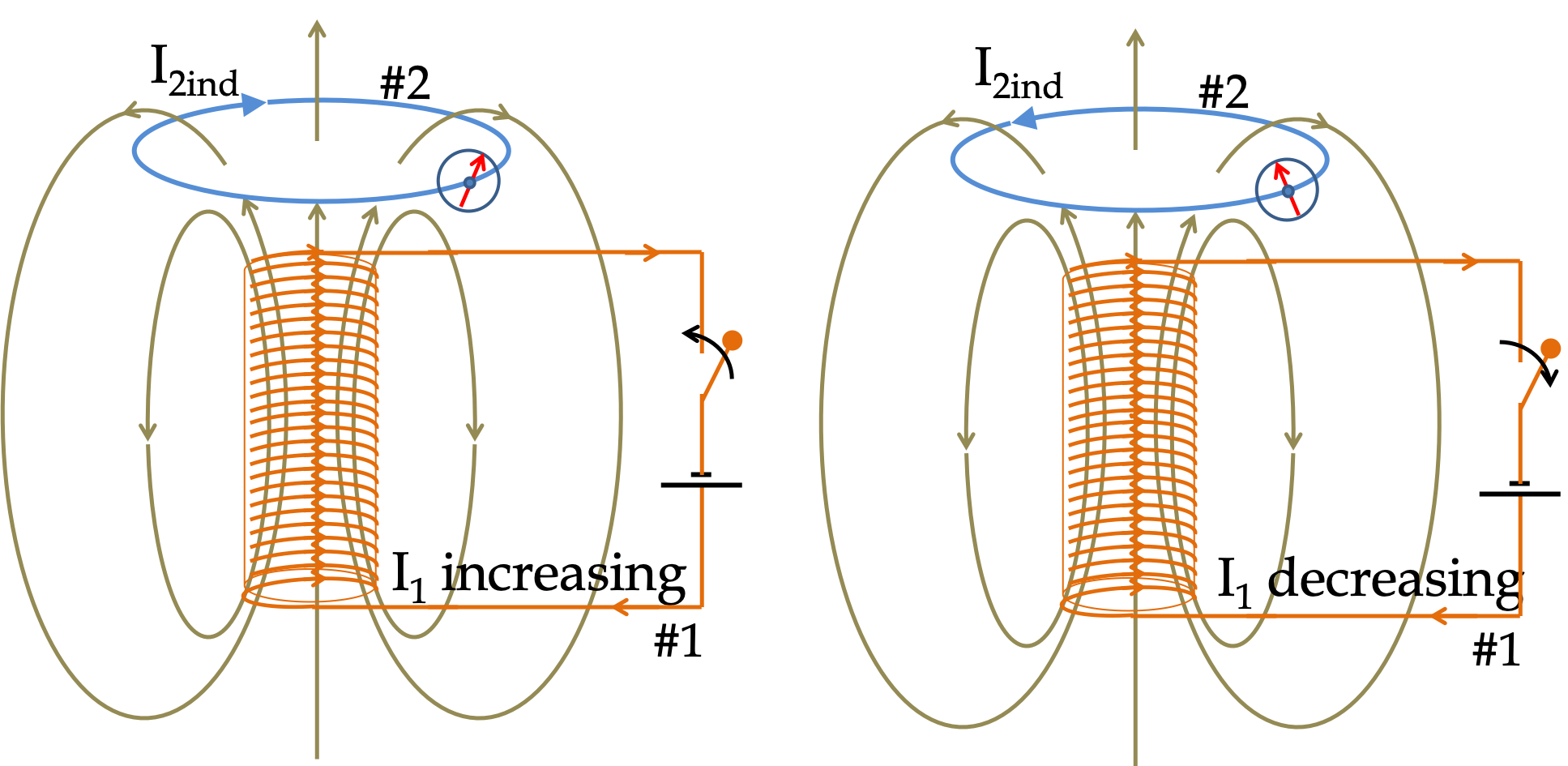